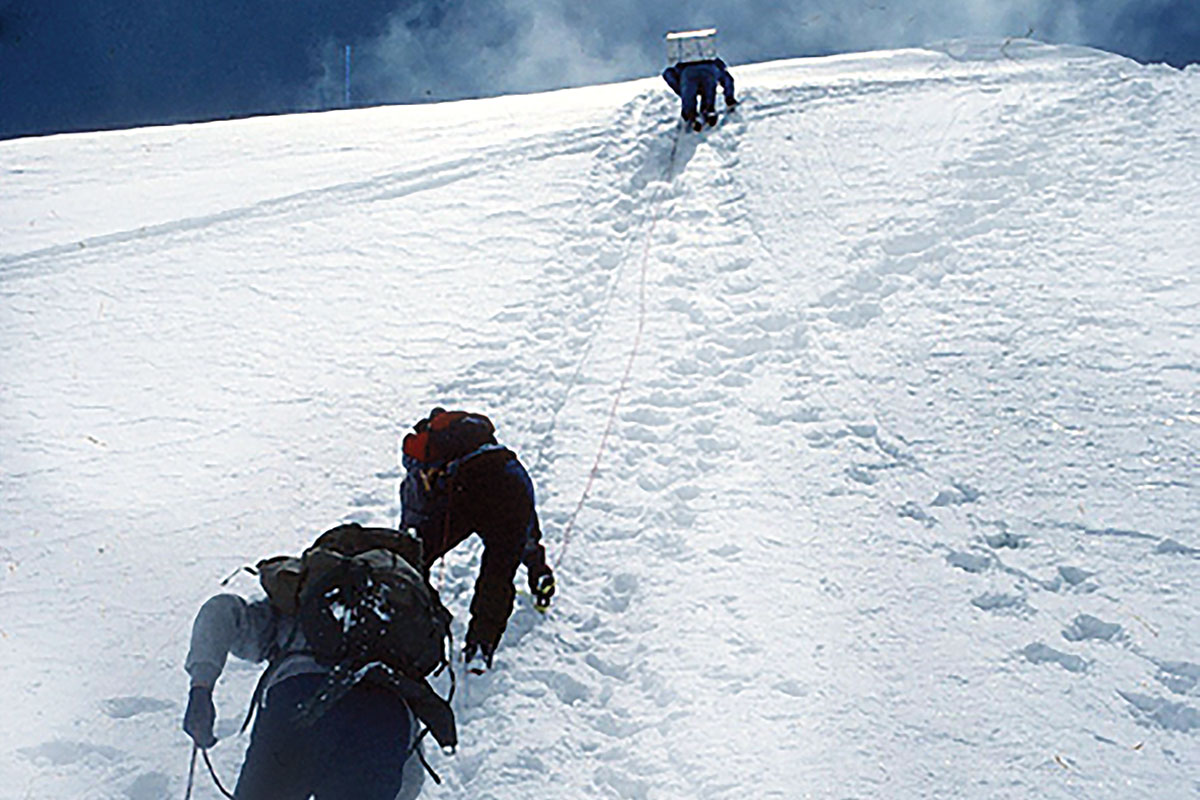
In a 1987 expedition, neurologist Marcus Raichle, MD, climbed 18,000 feet above sea level, scaling the icy Karakoram Mountains of Pakistan. There, amidst heavy snow and high winds, Raichle and 18 researchers injected themselves with radioactive xenon.
A crude scanner measured the gas as it diffused through their bodies, accurately recording blood flow in the mountaineers’ brains. Their objective was to better understand acute mountain sickness, a syndrome affecting climbers that causes headaches, vomiting, cerebral and pulmonary edema and, occasionally, death.
The British expeditionists’ earlier treks already had linked the syndrome to the brain’s uptake of oxygen. On this journey, they invited Raichle, an experienced mountaineer and physician highly regarded for his expertise in mapping brain blood flow.
Thirty years later, Raichle is still unraveling clues to the brain by studying its blood flow and oxygen use. This blood flow — laden with oxygen and nutrients — fuels the brain, with the busiest areas burning through fuel the fastest.
Raichle, now 81, the Edith L. Wolff Distinguished Professor of medicine and a professor of radiology, neurology, neurobiology and biomedical engineering, is a central figure in the history and science of brain imaging. He is noted for developing positron emission tomography (PET) techniques, explaining principles underlying functional magnetic resonance imaging (fMRI) and capturing some of the first snapshots of the brain at work.
He tells a thrilling tale of scientific mysteries and their startling solutions, which have transformed how we understand ourselves.
New imaging techniques
“If you were to write a history of brain imaging, there’d be a pop-out box about Marc Raichle at multiple spots,” said Steven Petersen, PhD, the James S. McDonnell Professor of Cognitive Neuroscience in Neurology and longtime collaborator. “Every five or 10 years, he’s done something seminal.”
Raichle joined the School of Medicine faculty in 1971, just before the science of brain imaging took off. Michel Ter-Pogossian, PhD — a physicist who would develop the PET scan a few years later — recruited him. They met at a scientific conference in London. Raichle had studied brain blood flow as a postdoctoral researcher, and a clerical error had resulted in a conference invitation being issued to him instead of his adviser.
At the time, Raichle was serving as a major and flight surgeon at the U.S. Air Force School of Aerospace Medicine in Texas, pursuing his interest in brain blood flow and screening pilots who had experienced medical problems impairing their ability to fly. Never one to turn down an opportunity, he hopped a military plane and attended the conference.
“Every five or 10 years, he’s (Raichle) done something seminal.”
— Steven Petersen, PhD
“There was no imaging at the time, but Ter-Pogossian had a detecting system to measure the regional oxygen metabolism of the brain, and he said he’d build me an even bigger, better one if I came to WashU after I finished my military service,” Raichle said. “So I said to my wife, Mary, ‘Let’s do this. This could be fun. We’ll stay for a couple years and then go on home to Seattle.’”
Soon after Raichle arrived in St. Louis, Ter-Pogossian and others at the medical school designed and built the first PET scanner and used short-lived radioactive materials called radiotracers to map organ function in living animals including humans. Raichle’s job was to develop protocols and algorithms to apply the new imaging technique to the human brain. Over time, he figured out how to separate important activity in the brain from background noise, and how to align images of different people’s brains so corresponding areas could be matched up and studied.
Today, PET scanning is widely used to detect tumors and metastases in cancer patients, evaluate the effects of a heart attack, measure brain activity and for many other purposes.
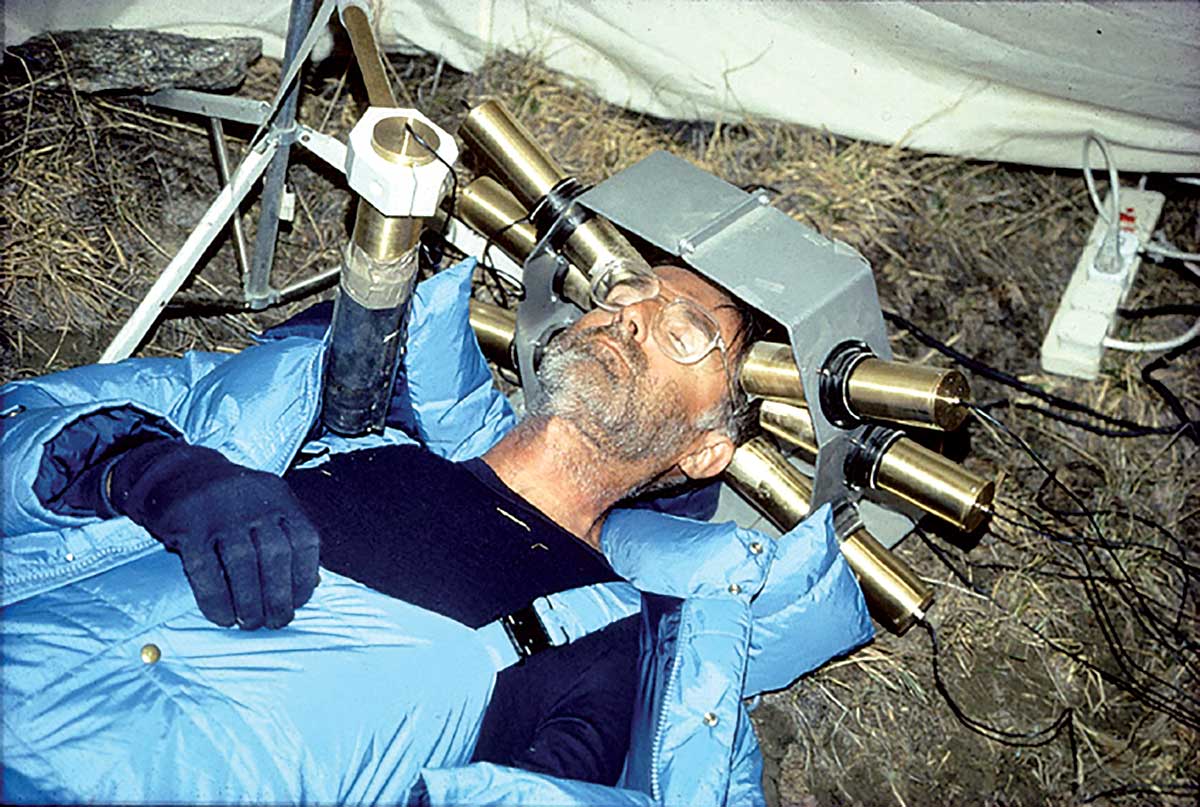
In 1988, an editor at the prestigious journal Nature called to ask about a project that Raichle, Petersen, cognitive psychologist Michael Posner, PhD, and others were working on: visualizing language processing in the brain. “In my long career, it is the only time Nature has ever called me up and said, ‘We hear that you’re doing X and we’d be interested in publishing it,’” Raichle said.
Using PET, the team monitored patterns in people’s brains as they read a word — hammer, for example — spoke the word, heard the word, and finally associated it with another word — such as nail.
By comparing the images, the researchers zeroed in on the brain areas that were active at each step. Put boldly, they took snapshots of the brain thinking.
“Marc opened up the human brain to scientific analysis,” Posner said. “It used to be that most of the understanding of brain physiology was based on nonhuman animals or on people with brain injuries. The idea that you could analyze human brain networks in normal, living people? That was just a dream. It wasn’t possible. Marc more than anyone else made it possible.”
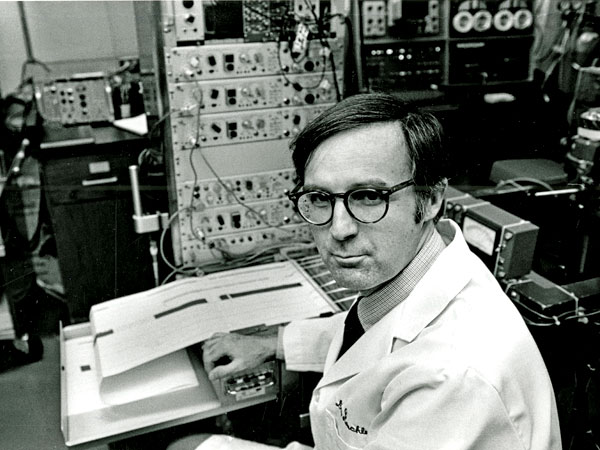
Raichle built his own lab near the Ter-Pogossian lab. Eventually, their two labs at Washington University’s Mallinckrodt Institute of Radiology (MIR) merged into the NeuroImaging Laboratories, now consisting of 33 faculty and over 100 trainees and staff.
A powerful cyclotron in MIR’s basement produces the radioactive materials researchers use for PET-based studies on topics as diverse as human cognitive and clinical neuroscience, human aging and neurodevelopment and brain physiology.
“When I came here 47 years ago, Michel had this vision that radioactive materials would be valuable in biology and medicine,” Raichle said. “The work we do here is quite sophisticated and technically challenging, but we take it for granted because, after all, it’s been going on at Washington University for nearly 50 years.”
A scientific firestorm
Raichle’s next major accomplishment was providing the data that explains how fMRI (functional magnetic resonance imaging) detects brain activity. And he was roundly castigated for it at the time.
For more than a century, physiologists had believed that blood flow and oxygen use rose and fell together. In other organs, they do. But the assumption had never been properly tested in the brain.
In 1986, Raichle and Peter Fox, MD, used PET scanning to show that when a part of the brain increases its activity, blood flow to that area increases but oxygen usage does not. This results in an increase in the amount of oxygen in active areas. It’s a case of supply increasing more than demand.
A few years later, Japanese researcher Seiji Ogawa discovered that changes in oxygenation are readily detected by MRI. In a sudden flurry of insight in the early 1990s, neuroimaging scientists put the puzzle pieces together — brain activity causes a change in blood oxygen concentrations, changing oxygen levels alter the magnetism of the blood, shifts in magnetism can be detected by magnetic resonance imaging — and fMRI was born.
Ogawa is rightly credited with founding fMRI, and Raichle and Fox had provided a key piece to the puzzle. But just a few years before, when their research was published, it had threatened to derail their careers.
“Prominent neuroscientists told Dr. Raichle that his ideas couldn’t be right and that he’d better correct the error or his career would go down the tubes,” said Avi Snyder, MD, PhD, a professor of radiology who has worked with Raichle for many years.
“People were almost throwing eggs at them,” Petersen said.
“But,” Snyder added, “the existence of fMRI proves that Marc was right and they were wrong.”
A noninvasive technique that does not require radiation, fMRI is now the most powerful tool we have to understand how the brain behaves. Researchers use fMRI to gain new insights into language, learning, emotion and memory — and to figure out how the brains of people with disorders such as schizophrenia or dementia differ from those of healthy people.
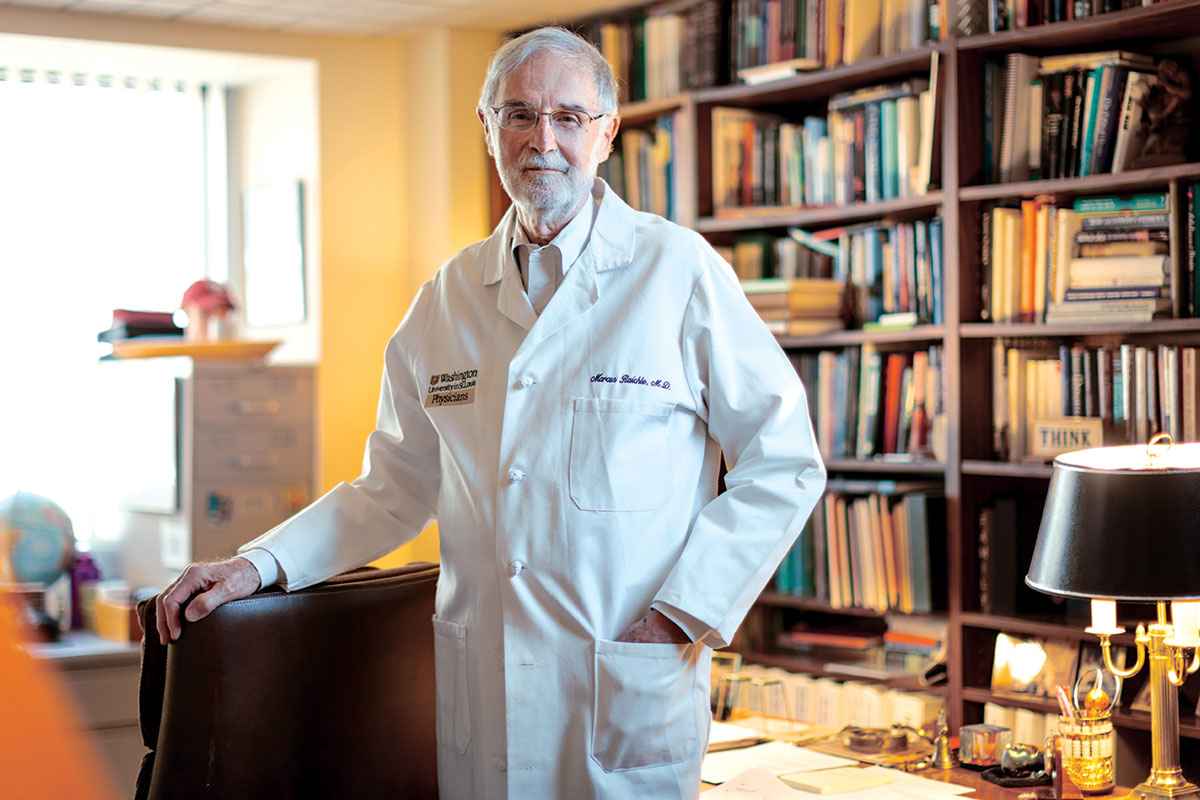
Hidden architecture
As the science of brain scanning progressed, researchers noted that the brain never quiets down. As a person lies quietly in a scanner, daydreaming, waves of activity pulse through his or her brain.
“Marc wasn’t the first to observe resting state activity, but he was the first to really understand that it’s not just noise,” Snyder said.
Philosophers and psychologists long have argued that the subconscious mind dwarfs the conscious one, but it wasn’t until researchers such as Raichle started measuring the brain’s energy use that there was hard data to back up their intuition.
It turns out that consciously performing a task only increases the brain’s energy usage by about 5 percent. Somewhere between 60 and 80 percent of all energy used by the brain goes toward activity seemingly unconnected to external events. Raichle dubbed this energy “the brain’s dark energy” and argued that it represented complex processes we are only beginning to understand.
“Most of what the brain is doing is nonconscious,” Raichle said. “So often people say something ‘feels right’ to them and conclude that it must be true. But your brain just made that judgment for you, and the reason it did so is probably totally opaque to you.”
As he scrutinized the images of the areas that decrease their activity when people shift their attention to a task, Raichle realized something else: The areas weren’t random. The same collection of regions always seemed to be involved — in the middle of the top of the brain, just above the eyes and on either side near the back.
In a seminal 2001 paper, Raichle and colleagues showed that these discrete brain regions formed a functional network — the default mode network — that plays a critical role orchestrating the background activity of the brain. Disruptions to this system have been noted in people with Alzheimer’s disease and autism spectrum disorder.
Other researchers found that similar networks control all the major brain functions, including movement, vision and attention. Moreover, these linkages can be found even when they do not seem to be in use. If you undergo a functional MRI scan, a computer algorithm can analyze your brain activity and find the motor network, for example, even if you haven’t moved or even thought about moving during the scan.
The technique of identifying functional networks while at rest is known as resting state functional MRI, and it is used in neurosurgical planning. Doctors need to pinpoint precisely where critical brain functions such as language and motor function are located so they can plan a surgical route to spare them, as each person’s brain is slightly different. With resting state fMRI, patients need only spend a few minutes in a scanner to give surgeons the information they need.
“Without Marc’s foundational efforts there’s no way I would be taking resting state fMRI and applying it to brain tumor patients and epilepsy patients,” said neurosurgeon Eric Leuthardt, MD, PhD, who is working on incorporating personalized brain maps into neurosurgical guidance software.
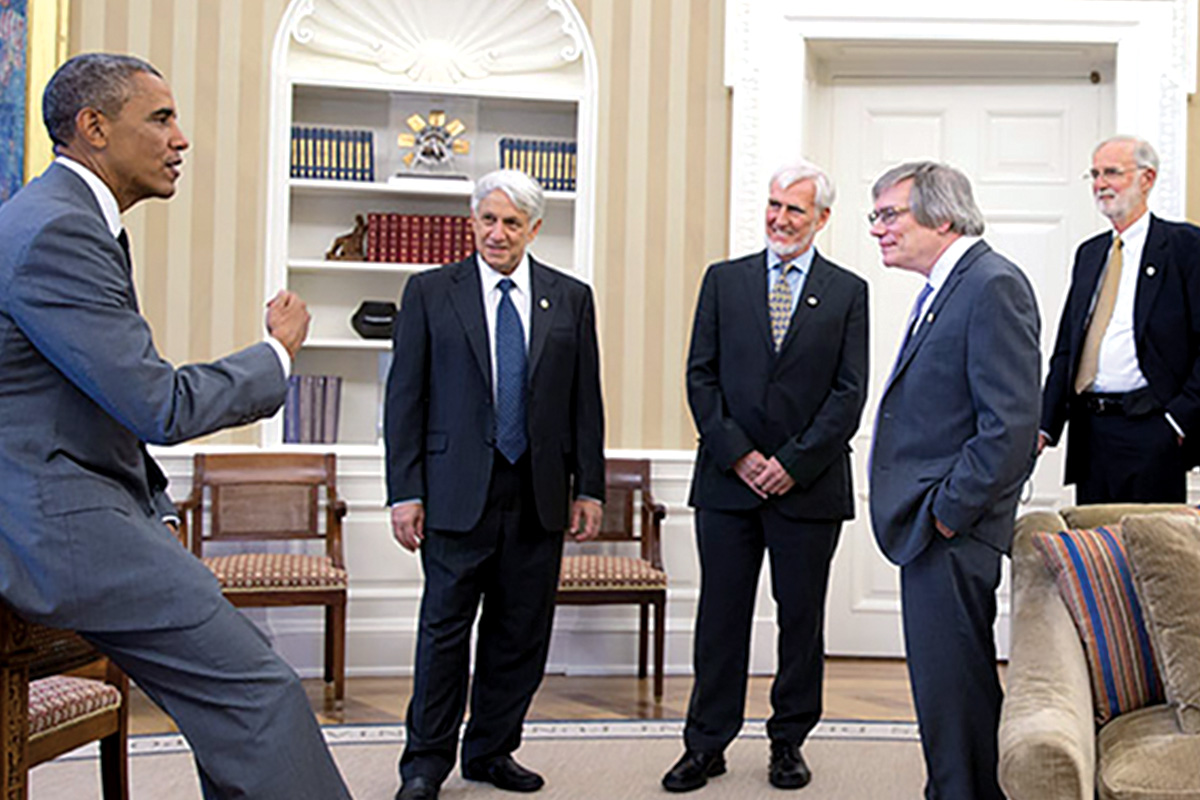
Raichle was awarded the prestigious Kavli prize for neuroscience in 2014, which garnered him a trip to the White House to meet President Barack Obama. In a half-century of work, Raichle has unearthed a hidden substructure of the brain, quantified the subconscious and brought the human brain into focus.
And he isn’t done yet.
Sugar: the next frontier
Raichle handed over management of the NeuroImaging Laboratories to Tamara Hershey, PhD, a professor of psychiatry and radiology, more than a year ago. But he still directs research projects from a corner lab office. Wooden shelves filled with books on every aspect of the brain line one wall, many of them written by friends and colleagues. To one side hangs a picture of Raichle on a Pakistani mountainside, bundled against the cold, triumphantly holding a homemade banner emblazoned with “Mallinckrodt Institute of Radiology.” In the midst of all this, Raichle — tall, slim and energetic — sits casually on the edge of a desk and talks about sugar.
“We’ve traditionally thought of glucose like coal: It arrives in cars to the brain and gets shoveled into the furnace to produce energy,” Raichle said. “But that’s only part of what happens to glucose in the brain. It’s also deeply involved in biosynthesis, neuroprotection and plasticity — the ability to form new connections between neurons as you learn new things.”
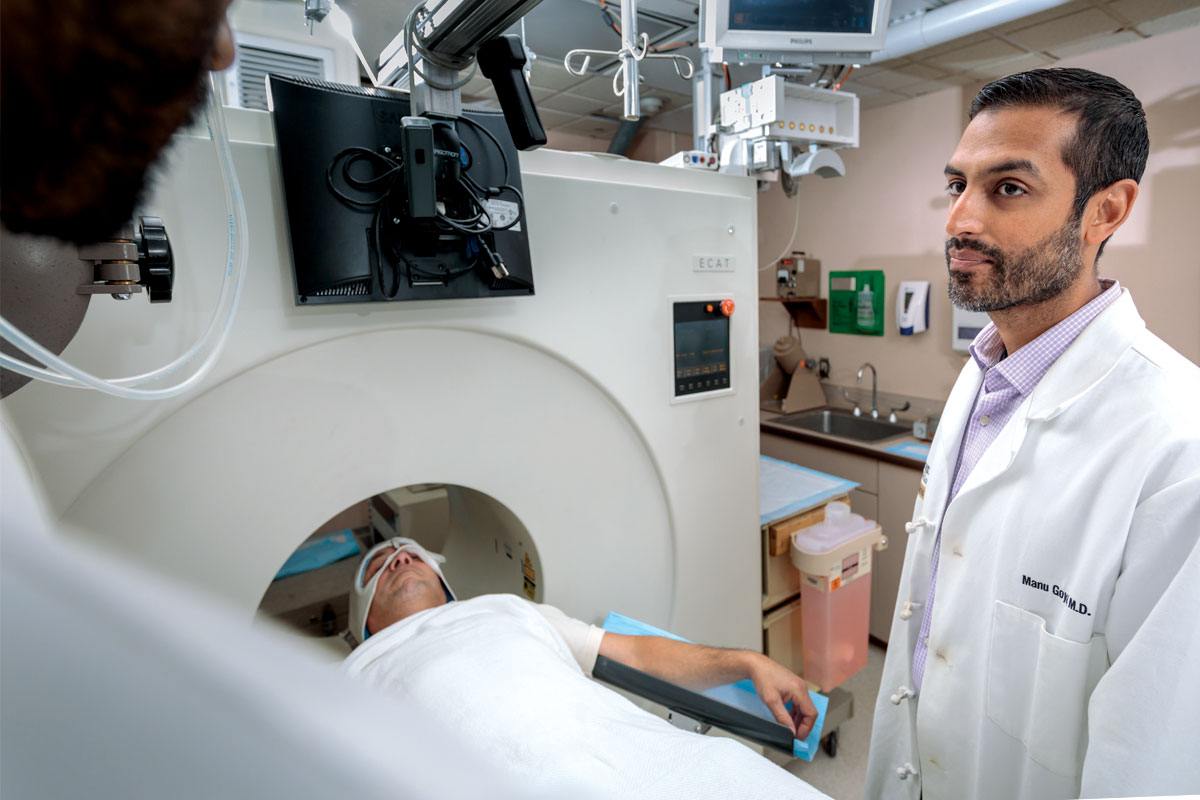
With Andrei Vlassenko, MD, PhD, and Manu Goyal, MD, Raichle is studying how the default mode network and glycolysis — the breakdown of glucose molecules — relates to aging and Alzheimer’s disease. The default mode network, which uses a lot of glucose, maps neatly onto the areas that degenerate most quickly in Alzheimer’s disease. With Hershey and others, Raichle is studying what effect high blood glucose levels have on glycolysis, and why diabetes is linked to Alzheimer’s disease.
“Marc has a unique ability to notice things other people overlook and then think deeply about what they really mean,” Hershey said. “He’s made revolutionary contributions to science already. His work with glycolysis is at a relatively early stage, but it may turn out to be his next major contribution.”
In addition to his research, Raichle travels frequently to the Washington coast where he and his wife both grew up. They own two houses there, with room to host their four children and 12 grandchildren. He thinks often of the promise he made to his wife 47 years ago to move back.
When he does retire, he will be missed.
“Marc’s stature in the field and scientific creativity draw people to him, but his kindness and generosity keep them there,” Hershey said. “I think we get all tied up in celebrating accomplishments, but to also be a wonderful person is really remarkable.”
Published in the Autumn 2018 issue